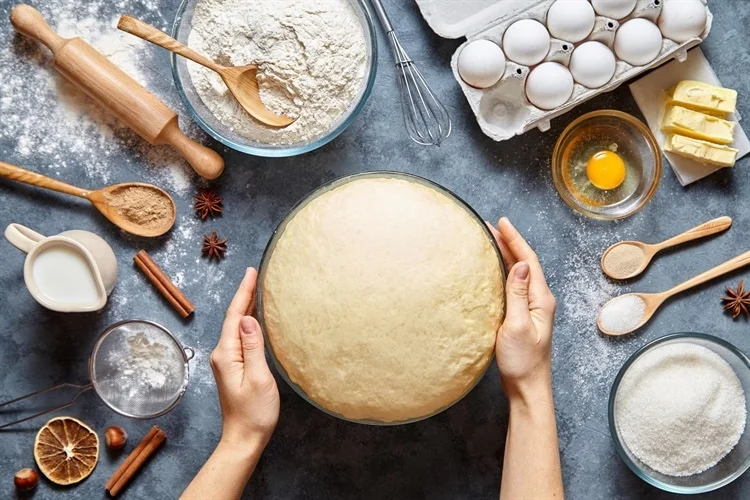
The Chemistry of Baking
Baking is certainly more niche than other, more popularized forms of food production. However, according to research performed by the National Purchase Diary Panel (NPD), 82% of all meals in the United States are prepared within the home- and a hearty amount of these require the use of an oven or other dry heating apparatus. This culinary practice is commonplace and requires a great deal of energy and planning to execute.
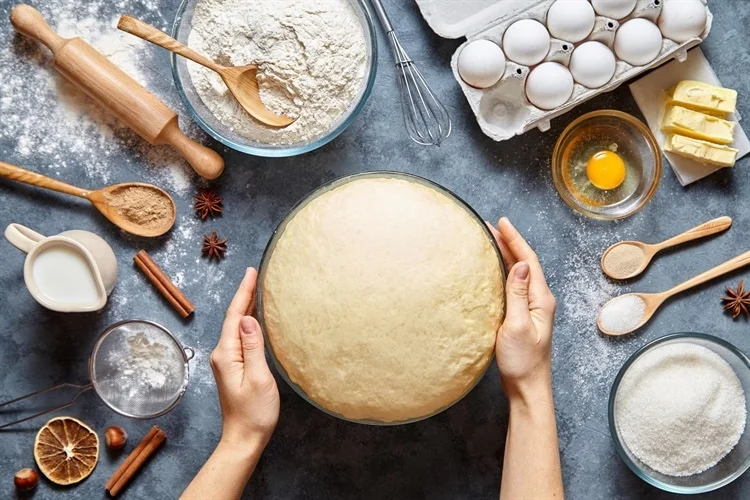
For these reasons, new advancements have been made in the field of baking. Baking research itself is growing proportionally to the popularity of this pastime.
Chemistry Based Improvements on Baking Methodologies
Baking itself requires a substantial amount of energy to perform, and some labs have been exploring more conservative mechanisms. In fact, approximately a third of all energy provided by a traditional oven goes into the dry heat cooking process, while two-thirds dissipate into the surrounding environment.
To make this process more energy-efficient scientists have been looking towards gas-fired heat sources that are radiative and can be condensed to a smaller area. Porous volumetric ceramic burners (VCBs) are just one example and may lead to a quicker baking time, as well as a reduction in energy input.
Gaseous water is commonly added to the baking chamber, which will proportionally decrease the convection, heat conduction, and thermal radiation of the apparatus. By excluding water from the study, the porous VCB and dough in question will experience a 45% heat transfer of thermal radiation. However, excluding water will also reduce the heat of conduction by 20%. By exploring these heat exchange mechanisms, baking can be optimized, and the issue of excessive energy thruput can be ameliorated.
Thermal analyses also regard pathogens like Salmonella or Listeria Monocytogenes. These species can survive under these dry environments, and the displacement of these microbiomes is critical when constructing thermal inactivation parameters.
Heating Contribution to Flavor Profiles
During the baking process, the loss of aromatic intensity is directly proportional to the temperature of the oven. The Maillard reaction is the culprit of this loss in aromaticity, by releasing these fragrant, volatile compounds into the environment. Under high temperatures, the sugar combines with amino acids within the dough’s proteins, releasing melanoidins as well as other organic compounds, that flow through the crust. This is what causes the dough to rise, and what causes the brown pigmentation atop the surface of the crust.
This loss of aroma, and tangentially flavor, is both inevitable and governable. Studies performed by Pico, J et al., and others like it, explore different forms of grain and their volatile release patterns using proton transfer-time of flight-mass spectrometry (PTR-ToF-MS). They found that toasting the bread matrix released these volatile compounds, though gluten-free breads presented greater losses than those of white bread. This could explain why gluten-free bread typically has a fragile, fainter aroma.
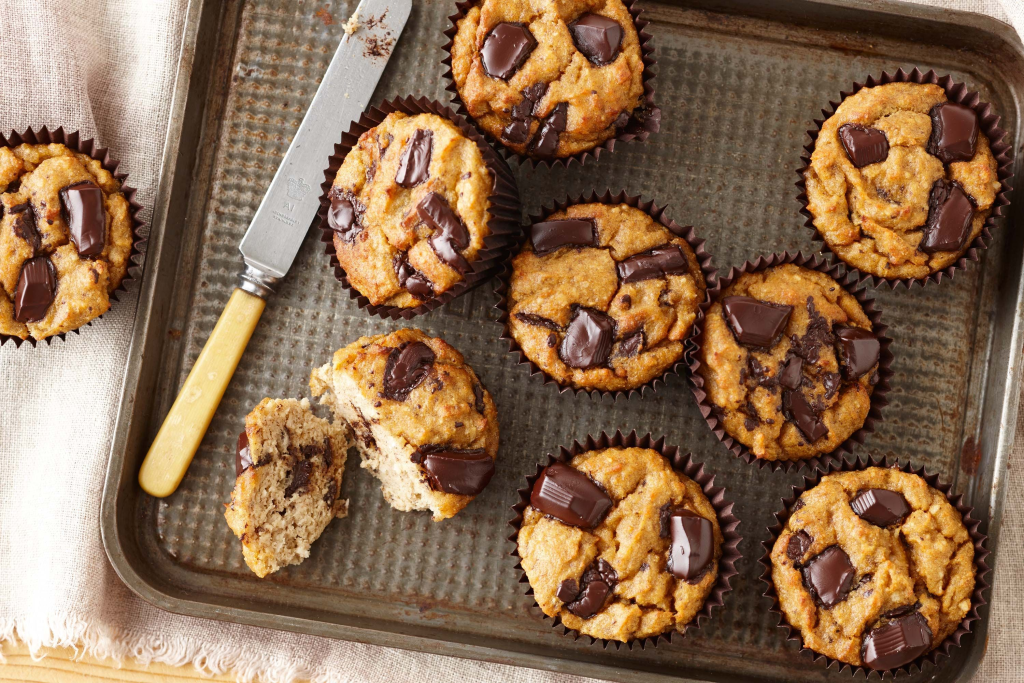
Salt, Flour, Yeast, and Water Interactions
The flour that we use in our dough contains both Gliadin and Glutenin proteins, this unity is what is typically referred to as gluten. Once exposed to an aqueous environment, these proteins will form both hydrogen bonds and disulfide bridges with one another. This will stabilize the tertiary structure of these higher-ordered proteins.
By the process of kneading, a cycle of severing and subsequent reforming of disulfide bridges will initiate. This redox reaction will give rise to the glutenin chain network and establish cohesive gliadins (globular proteins). The relaxation and exerted tension on the dough network will work the glutenin, which is what gives rise to its elastic properties.
Salt should always be incorporated into the baking medium. This is because the sodium and chloride ions will contribute to the cohesive nature of the protein chains, which leads to the dough’s texture and viscosity. This aggregation occurs because the hydration barriers between the gluten are disrupted as the water flows into the bulk solution, migrating from the surrounding proteins.
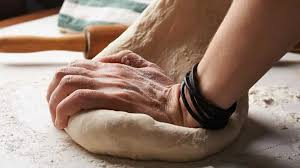
Baking at the Micro Biotic Level
The yeast Saccharomyces Cerevisiae, characteristically known as baker’s yeast, is what humans have used in the fermentation of many consumables for thousands of years. The 63 isolates that have been discovered, harvested from both fauna and flora, have had a profound impact in all alcoholic beverages, in most staple foods, and to a similar extent, bakery.
The S. Cerevisiae that accompanies this process will feed on maltose disaccharides provided by starch. This “sugar cycle” originates with amylase breaking down starch into maltose within the flour, followed by the subsequent breakdown performed by maltase in yeast to yield glucose.
S. Cerevisiae is the principal yeast strain in cooking, due in part to its great versatility. This yeast can tolerate numerous processing conditions such as freezing and drying while withstanding osmotic stress. It can utilize maltose and convert it to glucose with superlative efficiency. It accomplishes all this while still retaining the desired tastes and aromata.
Though S. Cerevisiae is the starter strain for most baking, the diversity of this eukaryotic microbe is still unknown. In addition, the microbial community used in baking can vary between grain type, geography, culture, and a surfeit of other factors (Calvert, M et al., 2018). Therefore, a great deal of research is being conducted on baking methodologies. Although we as humans have been baking for hundreds of years, the field itself may yet be in its infancy.